The joke was almost as long as the dream was: Nuclear fusion technology is about 30 years away… and will still be. But now, more than 80 years since Australian physicist Mark Oliphant saw Deuterium atoms first fuses and releases energy dollops, it is time to finally update the punch line.ore than two dozen research groups — beautifully staffed and well-funded start-ups, academic initiatives, and corporate ventures — have made significant progress on controlled nuclear fusion over the last few years. They create power using fusion reactors based on radically different designs that contradict the two conventional methods, which either use a huge magnetic vessel with a body shape known as a tokamak or a huge laser.

In addition, some of these groups expect major fusion power milestones in the next five years, including the breakthrough point at which the emitted energy overcomes the energy used to generate the reaction. That’s surprisingly soon, as traditional tokamak-based mainstream ventures and laser approaches work for decades and spend billions of dollars without breaking through.
In Cambridge, Mass., researchers from Commonwealth Fusion Power Systems associated with MIT say their new reactor concept exceeds that of 2025. In the United Kingdom, a spin-off called First Light Fusion University in Oxford claims to show its breakthrough in 2024. And TAE Technologies, a startup that has produced a breathtakingly ambitious five-year deadline for commercializing its fusion reactor in southern California.
Irrational lushness? Maybe, Fusion power science is one of the most expensive efforts, relying on high cash inflows just to repay energy bills in a laboratory. The tentation to overestimate potential accomplishments is high in the search of funding. And past hopes of imminent breakthroughs have been consistently shattered. Now, progress in high-speed computing, materials science and modelling and simulation has changed to overcome once recalcitrant technological barriers, and large sums of money pour into the field.
Some of the recent fusion power experiments are working to better understand and tweak the behaviour of ultra-high-temperature plasma in which hydrogen nuclei fuse to form helium. Others reopened promising investigative lines that had been shelved decades before. Others also use new superconductors or hybridise mainstream ideas.
Many of these new power projects would fail despite their strong resources and innovative approaches. But if only a reactor can be built that can economically produce electricity, it can change the path of human civilization. A single gramme of the most widely used hydrogen isotopes can theoretically generate the same energy as 11 metric tonnes, in a fusion reaction for power generation, helium as the only permanent by-product.
As climate change accelerates and energy demand soars, nuclear fusion promises a low-carbon, low-waste baseload power source that is relatively clean and free of the possibility of collapse or armament. This tedious prospect has kept the idea of fusion power alive for decades. Will one of these scrappy entrepreneurs finally make fusion power a reality?
Magnetic-Confinement Fusion (MCF)
Not long ago, the outlook for fusion power was quite dull, with apparently two of the major projects stalled. In 2016, the U.S. Ministry of Energy acknowledged that its National Ignition Facility (NIF) of US $3.5 billion failed to achieve the target of using lasers to “ignite” an autonomous fusion power reaction. A DOE study proposed [PDF] that NIF research should shift from laser ignition research to whether such an inflammation is even feasible.
The same year, the United States and several other governments started discussing whether the international thermonuclear experimental reactor could help them (ITER). ITER was first conceived in 1985 and is now being built in southern France and is the biggest fusion power experiment in the world. It is a form of tokamak which uses magnetic forces to contain and isolate the ferociously hot, energetic plasma necessary for fusion to start and maintain.
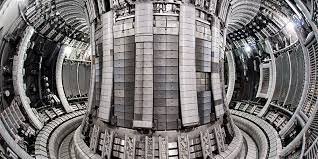
However, the project has experienced delays and cost overruns, which have quintupled its initial $5 billion price date to 2035. (And even when this date is set, decades after that before the design-based commercial plants are in operation.) The losses and immense expenses of NIF and ITER have not only drained capital but also excitement.
Even as the megaprojects for power that were supported by the government were established, alternative research on fusion energy began to gain traction. The hope of those who make these new attempts is that their new and smaller methods will accelerate the gradual slog that has gone on for decades.
Finally, investors take note and pump capital into the field. In the last five years, private capitalists have invested approximately $1.5 billion in small fusion energy firms. Amazon’s Jeff Bets, Microsoft’s Bill Gates, and the venture capitalist Peter Thiel were among those who made important bets on fusion power. Some major companies, including Lockheed Martin, have started their own small fusion ventures.
He said in 2016, Jesse Treu, scientist and physicist who has been investing a large part of his career in biotech and medium-scale startups, “wonderful things have begun to happen in fusion power, but funding has not caught up. Private equity and risk capital are obviously part of the solution to improve this technology, which is the best energy cum power solution for the world.” He co-founded the Stellar Energy Foundation to link fusion researchers to sources of funding and to support and advocate.
And public funds have begun to follow in a private way: the U.S. energy department, which has been using the majority of non-defense fusion allocations to ITER for decades, is now channelling some support to projects on the fringes of conventional science. The federal budget includes an increase of $107 million for fusion projects in the 2020 financial year, including research partnerships which enable small companies to carry out major experiments in national laboratories of the DOE.
The revived interest of the US government is partly due to a perceived need to keep abreast of China, which recently resumed its fusion energy programme, after a three-year moratorium. In Sichuan Province, the Chinese government plans to upgrade to a new fusion reactor this year. Meanwhile, Chinese energy firm ENN Energy Holdings has invested in research programmes abroad and, with the aid of top US scientists, is constructing a duplicate of Princeton Fusion Systems’ compact reactor in central China.
“Now it looks like China can engulf any idea that the United States fails to finance,” Matthew J. Moynihan, a nuclear engineer and investor fusion advisor, says, “that serves as a wake-up to the U.S. government.”
Inertial-Confinement Fusion (ICF)
Fusion power remains as difficult as ever with all this operation and expenditure.
For power, a fusion reaction occurs when the nuclei of a lightweight element, usually hydrogen, collide with sufficient power to combine and create a stronger element, contrarily to nuclear fission in which a large unstable nucleus is broken into smaller components. In the process, the mass is released and converted into energy, as described in the popular formula of Albert Einstein: E = mc^2.
There is an abundance of fuse energy in our universe—the sun and other stable stars are powered by thermonuclear fusion—but it’s probably the most challenging engineering challenge human beings ever have triggered and controlled a self-sustaining fusion power response and exploiting its strength.
In order to melt hydrogen nuclei, earthbound reactor designers must find ways of overcoming the mutual repulsion of positively charged ions, the Coulomb force, and bringing them near enough to bond via what is known as the strong nuclear force. Most approaches require such high temperatures — several orders of magnitude warmer than the central sun’s 15 million °C temperature — that matter can only be in the plasma state, in which electrons break free of their nuclear nuclei and propagate freely via gas clouds.
However, a high-energy plasma is notoriously unstable and difficult to regulate. It tears and wraps and tries to break free, and migrates to the edges of the ground, where it easily refreshes and disappears. The biggest problem around plasma fusion is how it is heated, how it is contained, how it is shaped and regulated. The two key methods are magnetic containment and inertia. Magnetic reactors like ITER try to keep the plasma in a tokamak with the use of strong magnetic fields. Inertial containment methods, such as NIF, usually use lasers to compress and implode the plasma so rapidly that it remains sufficiently long to react to it.

Scientists have long believed that more is better if stable and energy-dense plasma fields are to be formed. But with recent developments in supercomputing and complicated modelling, researchers reveal more of the mysteries of plasma conduct and learn new tricks to manage them without massive, complex machinery.
Physicist C. Wendell Horton Jr. of the University of Texas Institute of Fusion Studies is one of the leading researchers in this work. He uses the Stampede university supercomputer to create simulations of plasma flow and turbulence inside magnetic containment reactors. “We’re making calculations only a few years ago that were impossible and modelling three-dimensional, time-consuming plasma data,” Horton states. “We can now see what’s going on even more nuanced and detailed than with analytical hypotheses and even the most advanced tests. This gives us a more complete image of what is needed to enhance the design of the reactor.”
The results of Horton have influenced both the design of large-scale experiments like ITER and small-scale projects. “The issue with ITER is that they have not worked out how to react to the plasma, however well they behave,” he says. “It will always flame out in a few minutes, and that obviously doesn’t solve the energy issue.” He and other researchers agree that some of the small attempts are far closer to a stable reaction that could produce baseload electricity.
TAE Technologies from California (formerly Tri Alpha Energy), founded in 1998, is one of the most mature fusion startups.
The TAE reactor is designed to use the so-called field-inverted (FRC) configuration to create a spinning plasma ring which is in its own magnetic field. (The architecture of Princeton Fusion Systems is also an FRC.) Another example is the TAE reactor, which injects beams of high-energy neutral hydrogen particles into hydrogen boron fuel instead of using deuterium and tritium — a hydrogen isotope mix that fuels the majority of fusion reactors (ionized helium nuclei). The heat generated by soft X-ray energy in the containment vessel is converted to electricity through a traditional heat conversion device, which heats water into steam to power the turbine.
Hydrogen-boron fusion is aneutronic, so that no harmful neutron radiation results from the primary reaction. The disadvantage is that combustion needs exceptional temperatures up to 3 billion °C. “The electrons radiate like crazy when you are that wet,” says William Dorland, Professor of Physics at Maryland University. “They’ll cool the plasma more quickly than you can heat it.” While FRC machines seem less likely to be plasma instabilities than some other magnetic containment procedures, an FRC reactor which can produce stable plasma has not yet been demonstrated by anybody.
TAE cofounder and CEO Michl Binderbauer states: “The new system, called Norman, is achieving substantial improvements in plasma containment and stability over the previous generation of machine” in honour of company cofounder Norman Rostoker.” The advances in artificial intelligence and machine learning, allowed by Google’s advanced algorithm called Optometrist, are driving the progress. In collaboration with Google, TAE has adapted the algorithm to analyse the data on plasma activity and to analyse the combination of variables that generate ideal conditions for fusion. The researchers identified this in a 2017 Nature article.
“We do things that we never could do ten years ago and that leads to faster and faster learning times,” says Binderbauer.
Magnetized Target Fusion (MTF)
Advanced computing also gives new life to ambitious research lines which were abandoned years earlier by budget cuts or technological roadblocks. The Canadian plasma physicist Michel Laberge founded General Fusion, based near Vancouver. He stopped working profitably to build laser printers to follow a magnetised target fusion method (MTF). The business has attracted more than 200 million dollars including investments by Jeff Bezos, Canadian and Malaysian governments.
The design of General Fusion for power combines magnetic containment and inertial containment fusion characteristics. The plasma fuel is injected into a sphere filled with a vortex of molten lead and lithium. Pistons around the reactor accelerate the shock waves into the core, compress the fuel and force the particles to fuse. It absorbs the heat in the liquid metal and uses it to create steam to rotate a turbine and generate electricity.
“You should somehow think of it as opposite to a tokamak,” Laberge says. “Tokamaks operate with a [relatively] low-density large plasma region. We are attempting to produce a mini-plasma of extreme density by squashing it with the waves of shock. Because the field is so dense and thin, we just need a millisecond to hold it together to react.”

In the 1970s, the U.S. Naval Research Laboratory experimented with a nuclear fusion piston device. These experiments failed because the timing of the shock waves could not be precisely regulated. The Laberge team has developed advanced algorithms and high-precision control systems for the speed, timing and compression of shock waves.
“The issue was symmetry in those experiments in the 1970s,” says Laberge. “Now we have achieved the precision and strength we need to resolve this section.”
Field-Reversed Configuration (FRC)
The use of liquid metal could overcome another of the main challenges of fusion energy: Radiation from neutrons erodes the walls of a reactor and must regularly be replaced and removed as low-level radioactive waste. The liquid metal protects against damage to the solid exterior wall. The liquid metal is irradiated, so there is no need to replace it periodically and therefore the reactor does not emit a constant flow of low level waste.
The newest plasma generating reactor, General Fusion power, for the first time in the end of 2018, is the core of the plant, Laberge says, showing end-to-end capability to generate nuclear fusion electricity. “Now that we’ve successfully produced a long-lasting, stable plasma, we can see we’ve got a viable way for plasma to produce more energy than it absorbs,” he says. “Our timeframe is now a matter of years, not decades, in terms of marketing.”

HyperJet Fusion Corp. based in Virginia has a solution similar to General Fusion, but rather than pistons, 600 plasma weapons shoot plasma jets into the reactor. The jets merge into a plasma shell or liner that implodes and then ignites a magnetised plasma target. The machine does not require a heating system to get fuel to fusion temperatures, says HyperJet CEO and F. Douglas Witherspoon, the chief scientist. “The plasma imploding liner holds the plasma target and provides the energy to raise the temperature to fusion conditions. And when we use a plasma that is much higher than a magnetic containment device, it decreases from metre to centimetre the fusing plasma.”
Witherspoon says that the HyperJet method has advantages over tokamaks that costly superconducting magnets are not needed to produce the enormous magnetic fields required to limit the combustion plasma.
Tokamaks themselves also reboot with various superconducting materials, which would make magnetic containment more viable. MIT’s spin-off Commonwealth Fusion Systems uses a high-temperature supranational yttrium-barium-copper oxide (YBCO) in its Sparc reactor.
Martin Greenwald, Co-founder of the Commonwealth and Deputy Director of Plasma Science Center MIT, estimates that the YBCO magnets of the Sparc reactor will be able to produce a chamber of around 21 teslas at their surface and 12 T at the centre of the plasma that approximately doubles the field strength of the niobium-tin magnets. Stronger magnetic fields exert more restraining strength on the charged plasma particles, improving isolation and allowing a much smaller, less expensive and possibly better performing fusion system.
“If you can double the magnetic field by half, with the same output, and cut the system in size, that will change the game,” says Greenwald.
Indeed, a benefit of the latest, smaller fusion ventures is that they can focus on new aspects of their designs and use decades of hard-won experience of the foundations of fusion technology. As Greenwald put it, “We think we can achieve fusion power plant commercial deployment faster if we embrace the traditional physics basis created around the ITER experiment and concentrate on our cooperation between physicists and magnet engineers who set records for decades.
Stellarator
However, some promising startups do not follow conventional wisdom and tackle the fundamental physics of fusion in new ways. The First Light Fusion is one of the most radical methods. The company intends to manufacture fusion using a very noisy crustacean-inspired inertial containment reactor design.
The distinguishing characteristic of the pistol shrimp is its pistol-like claw, which is used to shock the prey. After the hammer is taken down, the shrimp snatches it against the other side of the claw and creates a rapid pressure shift that produces vapor-filled voids in the water known as cavitation bubbles. When these bubbles collapse, 25 metres a second shock waves drive through the water sufficient to remove small marine animals.
“The shrimp just want to use the wave to stun their prey,” says Nicholas Hawker, co-founder and CEO for First Light. “It does not matter that the steam in the cavity, when imploded, is compressed so strongly that plasma is formed – or produced the Earth’s only example of inertial containment fusion.” The plasma is over 4,700 °C and produces a bang of 218 decibel.
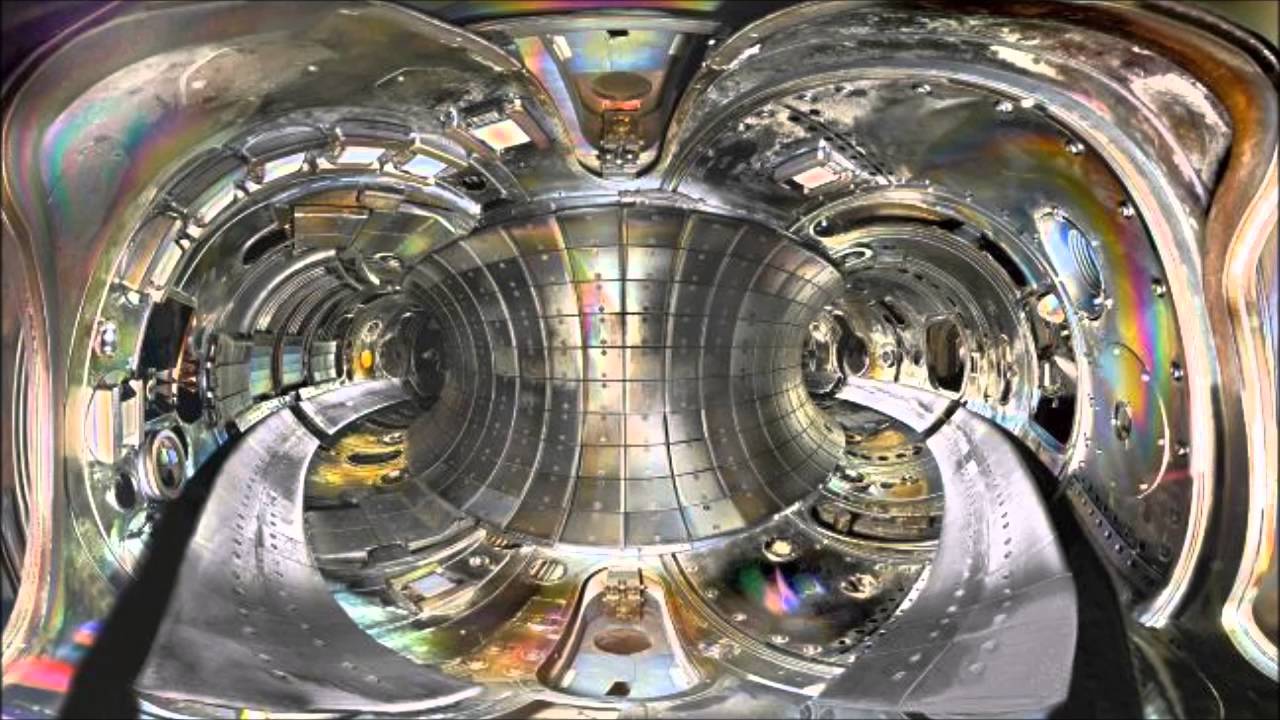
Hawker concentrated in his doctorate from the University of Oxford on the remarkable claw of pistol shrimp and started to research whether the physiology of shrimps can be imitated and expanded to generate a fusion reaction that can produce electricity.
After collecting £25 million (about 33 million dollars), and partnering with Mott MacDonald, the International Engineering Company is designing an ICF reactor that includes a metal disk-shaped projectile and a cube containing a cavity filled with deuterium tritium fuel. The impact of the projectile produces shock waves that generate cavitation bubbles in the fuel. As the bubbles collapse, the fuel in them is sufficiently compressed and forcefully fused.
Hawker says First Light expects its first fusion response this year and a net energy benefit by 2024. But he admits that those accomplishments will not be enough. “The energy of fusion does not only have to be theoretically feasible,” he says. “It must be economically viable.”
No one thinks it would be straightforward, but for many scientists and engineers who recently got into the arena, the extraordinary challenge of fusion energy — to mention the urgent need — is part of the attraction. And they have the money to fund their work more and more.
“The idea that fusion is another thirty or forty or fifty years away is false,” says Binderbauer of TAE whose company has raised over $600 million. “We will see the commercialization of this technology in half a decade’s time frames.”
Veteran fusion researchers like Dorland and Horton seem to have a more tempered perspective. They worry about great promises that fail, as has happened in the past, that can undermine public and investor support. Any marketing statements inside the decade “are simply not accurate,” says Dorland. “We’re very much more than a breakthrough from a road to fusion fuel.”
However, few argue about the desperate need for nuclear fusion in the near future.
“I believe it doesn’t go too far to claim that the moment of Kitty Hawk fusion is,” says Greenwald of MIT. “We haven’t got a 747 jet, but we ride.”
Also Read: Calculations for Fusion Reactors are now easier. AI Is All The Way Together
The Entrepreneurs Diaries is now available on Telegram. Join our telegram channel to get instant updates from TED.